Index
- Acknowledgements
- Introduction
- Theory behind Radiation & Radioactivity
- Radiopharmaceuticals – What, Why & How
- The Future
- References
- Bibliography
Acknowledgements
This article would not have seen its completion without Kvalito Consulting Group, my team, which assisted me in the writing process and enabled me to successfully publish it. Special thanks are also due to Dr Rafał Walczak, whose broad knowledge and experience proved to be an indispensable and invaluable support.
Introduction
It was estimated that from 2017 to 2020, around ten million people died annually from cancer [1-4]. It may be difficult to say if the trend changed or stayed the same throughout 2021 due to the Covid-19 pandemic, which influenced cancer diagnosis and treatment [5]. It is also predicted that the number of cases will increase in the future and, with them, the number of fatalities [6]. With such statistics and the fact that it is one of the leading causes of death in the world [3], cancer may be classified as a civilization disease [7] with one person for every six dying from it every year [4]. Of course, methods for its treatment exist, such as hormone therapy, surgery and chemotherapy, but with rapidly developing technology, potential and novel ways are bound to appear. One of the methods that may be described as a precursor of personalized medicine is nuclear medicine [8].
Nuclear medicine is a branch of medicine that uses radioactive elements, called radionuclides or radioisotopes, to diagnose or treat diseases. As the name suggests, its working principle is related to the nucleus of an atom and the characteristic of some chemical elements’ nuclei to emit radiation in the form of electromagnetic waves or particle ejection due to energetic instability.
When a radiopharmaceutical, that is, a drug containing a radioisotope, is introduced into the body of a patient by ingestion, inhalation or injection, it accumulates around a tissue or organ of interest and emits radiation. This process can be used to achieve one of the two goals – diagnosis (also called imaging) of disease or therapy (treatment). In the first case scenario, a special camera, such as in PET (Positron Emission Tomography) or SPECT (Single Photon Emission Computed Tomography) catches electromagnetic waves emitted from the patient’s body and creates a detailed image of structure, but also function of the target tissue or organ, including on-going chemical and biological processes. On the other hand, instead of capturing radiation through a camera, alpha and beta radiation, as well as Auger electrons, destroy targeted cells, resulting in the elimination of the lesion in the treatment of disease.
Many pharmaceutical companies focus on nuclear medicine as a method for diagnosis and treatment of diseases, mainly different types of cancer, for example, prostate or neuroendocrine cancer [9], and those that target other organs such as breasts, thyroid or the kidney [10,11]. Advanced Accelerator Applications, Precirix, Curium, Eckler & Ziegler and Radiopharm Theranostics are only a few examples of companies that specialize in the development of radiopharmaceuticals or the production of radioisotopes. Some of the products on the market include Lutetium-177, Gallium-68 [12], Iodine-131 [13] and Fluorine-18 [14].
The Theory behind Radiation & Radioactivity
Electromagnetic waves are composed of electric and magnetic fields, which are perpendicular to each other and propagate together in the same direction without the need for a medium – matter that waves travel through, like water or air. Electromagnetic radiation, which consists of those waves, is a form of energy. It can be depicted using an electromagnetic spectrum, which shows different types of radiation based on frequency (the number of waves per second, symbol: ν, unit: s-1/Hz) and wavelength (symbol: λ, unit: m).
Figure 1. [15] The electromagnetic spectrum. The energy of electromagnetic waves increases with a decrease in wavelength and an increase in frequency.
Electromagnetic radiation can be divided into two main categories, based on the amount of energy it carries. Non-ionizing radiation does not have enough energy to push out electrons from their atomic shells and, thus, is generally not as dangerous to human health. This type of radiation is commonly used in daily life, like in microwaves and radios. Light, which is in the visible part of the spectrum, is used in the phototherapy of infants [16]. People emit radiation from their bodies in the form of infrared radiation. However, the results of recent research state that prolonged exposure to infrared radiation may have serious consequences for the eyes and the skin [17,18].
On the other hand, ionizing radiation can pose a health risk if exposed to it for a long enough period. It carries sufficient energy to remove electrons from atoms and can thus cause serious damage, depending on the time of exposure and the amount of energy it has. In the order of increasing energy, X-rays and gamma rays are such examples.
Ionizing radiation is present in everyday life, and people are exposed to it in various doses, usually trace amounts. The sources can be natural, like cosmic radiation from space, Uranium and Thorium in soil [19] and Carbon-14 in the atmosphere [20,21], but also artificial (radionuclides produced in reactors, generators or cyclotrons, like Nickel-63). Radiation is a common occurrence, even if it may be difficult to observe and clearly understand its influence. For example, if a room is low-ventilated, radioactive Radon-222 can accumulate in it [22]. Some countries produce energy using nuclear reactors and there also exist nuclear bombs. Radiation is present everywhere.
Radioactivity is a characteristic of some chemical elements’ atomic nuclei to decay due to their instability in energy, mass, or both [23] and as a result, emit radiation in the form of electromagnetic waves (gamma radiation) or radiation in the form of particle ejection (alpha and beta radiation). There are many elements that have radioactive forms that differ only by mass number, that is, the number of neutrons in their nuclei, interchangeably called radioisotopes or radionuclides. For example, Yttrium-90 and Iodine-131 are used for medical purposes [24]. The non-radioactive, stable isotope for iodine is Iodine-127, whereas for yttrium, it is Yttrium-89.
Radionuclides have two main characteristics: a specific half-life, as well as the type and energy of radiation they emit.
Half-life (T1/2) is the time that it takes for half of the original quantity of a radioisotope’s nuclei to decay. Because a drug containing it is administered to a patient, it must have such a short half-life that it will not stay in the patient’s body too long, but also not too short, such that the radioisotope is able to release enough radiation to kills cancer cells. Different radioisotopes have different half-lives, for example, Plutonium-239 has a T1/2 of 24,100 years [25], whereas T1/2 of Lutetium-177 is 6.7 days [26].
The energy of a radioisotope can be expressed in electronvolts (eV). According to Kasperek-Nowakiewicz [27], a radiopharmaceutical best suited for medical usage is one which emits from 100 to 500 MeV (1×108 eV to 5×108 eV) of radiation energy.
The emission of energy may be in the form of electromagnetic radiation, such as the highly energetic gamma (γ) radiation, which is a form of energy with no mass, and thus has high penetration ability, or in the form of particle ejection, like alpha (α), beta plus (β+) or beta minus (β–) radiation, which carry even more energy than γ radiation and have mass, but their penetration ability is weaker. Usually, the former, including β+ radiation, is used for nuclear medicine imaging, whereas the latter is used for cancer treatment, since the amount of energy the ejected particles have can successfully break DNA bonds and thus kill the cells.
Figure 2. Types of radiation and their penetration abilities. Only an alpha particle can be stopped by a sheet of paper, as well as by the skin. Beta and gamma radiation, including neutrons, have higher penetration ability, thus, can only be stopped by using denser materials.
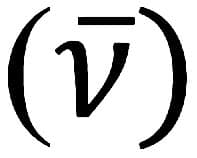
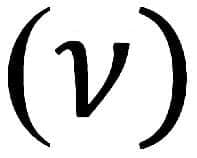
Actinium-225 is radioactive and decays by emitting an alpha particle. The following is thus an example of a nuclear equation for alpha decay:
When Actinium-225 (the parent atom) decays, it emits the nucleus of helium and thus its mass number decreases by four and its atomic number by two. As a result, only the α particle and the daughter atom, that is, an atom of francium is left.
The process is similar for β– and β+ decays, but the mass and atomic numbers of the parent atoms undergo changes under a different principle since the emitted particles are different.
β– decay of Carbon-14 (emission of the electron):
β+ decay of Carbon-10 (emission of the positron):
Radiopharmaceuticals – What, How & Why
A radiopharmaceutical is a drug that contains a radioactive isotope in its structure, but it is not its only component. The atom that emits radiation needs something that can guide it through the human body – a targeting molecule, also called a vector. Without it, the radioisotope would wander around the organism and accumulate in places where it was not supposed to find itself in. This targeting molecule can be a peptide, small organic molecule, protein like an antibody and many others [28]. There also needs to exist a linker between the vector and the isotope – a chelator, such as DOTA. Radiolabelling is a process by which a radioisotope is attached to a molecule [8] (a linker or a linker with an already attached vector).
Figure 3. [29] The overview of the structure of a radiopharmaceutical.
There are some radioisotopes that do not need a vector to arrive at their specified destination in the human body. Radium-223 behaves similarly to calcium – it can accumulate in the damaged bone areas caused by cancer [30]. Another example is Iodine-131, which is used for the treatment of cancer in the thyroid since most of iodine naturally gathers in that area of the body [31]. If there was a release of radioactive iodine into the environment due to a nuclear plant accident, as in 1986 in Chernobyl, Ukraine, taking big doses of Iodine-131’s stable counterpart may contribute to the prevention of the radioactive isotope’s accumulation in the thyroid [32].
Radioisotopes can be found naturally in the environment, but the ones used for the purposes of nuclear medicine are usually produced in nuclear reactors, generators or particle accelerators (e.g., cyclotrons). They are then taken to radiopharmacies, where they are transformed into radiopharmaceuticals, which are then transported to hospitals and introduced to patients by injection [8] or other means. When storing a radiopharmaceutical, the type of radiation the radionuclide emits must be taken into consideration. For example, if it emits gamma radiation (only, or with the addition of alpha and/or beta radiation), it must be stored in a container made from thick metal, like lead or iron (refer to Fig. 2), so that radiation cannot pass through it and cause harm to the external environment.
Vermeulen et al. [28] describe three ways in which radiopharmaceuticals achieve their goal of killing cancer cells – by the formation of chemically active free radicals, breakage of DNA bonds due to direct interactions with radiation or by an attack from the immune system of the patient. The key is to stop the replication process of the cells or to initiate their death [8].
A targeted radiopharmaceutical should theoretically act as a magic bullet that enters the body of the patient, accumulates in the targeted organ or tissue, kills off the tumour and leaves the body. More than a hundred years ago, Paul Ehrlich, a German scientist considered as the founder of chemotherapy, laid the foundations for the magic bullet concept based on his research. The magic bullet is a drug that goes straight to its intended target in the human body without inflicting any collateral damage to the surrounding tissues or cells [33]. It is supposed to behave like a programmed projectile. In nuclear medicine, this result depends on the vector chosen to specifically target an intended organ, tissue, or cells. The key is also the design process. A radiopharmaceutical needs to be designed in a way that, when it enters the body of a patient, it accumulates in the areas of the disease, not in healthy parts of the organism.
In reality though, nothing is perfect. The occurrence of short-term and long-term side effects is not impossible and does happen. Nausea, skin rash and tiredness are just a few of them. However, these symptoms are mostly due to the introduction of a drug, a foreign substance, into the body, not directly because of the radionuclide or radiation emitted by it.
Even after the targeted cells are successfully killed by the radiopharmaceutical, the radionuclide that does not emit alpha or beta radiation anymore can still accumulate in the body and emit gamma rays, which, on the other hand, are not necessarily harmful to the organism due to their high penetration ability. The accumulated radionuclide does not pose much risk, as it does not emit particle radiation, and the dose left in the body may not be toxic enough to cause harm.
The benefits outweigh the disadvantages. Treatment using nuclear medicine is employed when all other methods used beforehand, like surgery or chemotherapy, have failed or were not effective enough. In those cases, the benefits of nuclear medicine show their greatest advantage. For example, if cancer spreads to many parts of a patient’s body, targeted radioligand therapy (RLT) can be used to effectively kill the cells in those affected places.
The Future
Radiopharmaceuticals provide diagnostic and therapeutic solutions in medicine. They also have applications in analysing brain or heart activity and can aid in creating a diagram of how a specific substance spreads in the human body. Radionuclides themselves can also be used as indicators or tracers in technological processes.
Due to highly developing technology, nuclear medicine will become even more advanced as time goes on. The implementation of artificial intelligence, which is a big trend nowadays, may enhance the safety of doctors conducting diagnosis and treatment. Moreover, tasks that are currently difficult may become fully automated in the future, which will make them easier to carry out. The industry also carves a new direction in nuclear medicine, i.e., theranostics, which is a mixture of diagnosis and therapy that may turn out to be a more effective approach for patients.
What is even more important than the treatment of diseases, however, is their prevention. It may be difficult to achieve this goal using nuclear medicine. Instead, it may become possible to prevent diseases using genetic engineering in the future. Gene therapy works by introducing genes into the body that can aid treatment or replace/inactivate disease-causing genes [34].
Regardless of whether gene therapy develops to such a level that there would be no need to worry about treating diseases in the future, the perspectives of nuclear medicine are still broad, and only time will show how big of a change will occur in the upcoming years.
References
[1] Institute for Health Metrics and Evaluation. (2022) Cancer deaths rose to 10 million worldwide in 2019, [online], available: https://www.healthdata.org/news-release/cancer-deaths-rose-10-million-worldwide-2019 [accessed 30 November 2022].
[2] National Cancer Institute at the National Institutes of Health (2020). Cancer statistics, [online], available: https://www.cancer.gov/about-cancer/understanding/statistics [accessed 30 November 2022].
[3] Roser, M. and Ritchie, H. (2015) Cancer. Our World In Data (project of the Global Change Data Lab), [online], July, available: https://ourworldindata.org/cancer [accessed 30 November 2022].
[4] World Health Organization. (2022). Cancer, [online], available: https://www.who.int/news-room/fact-sheets/detail/cancer [accessed 30 November 2022].
[5] American Cancer Society. (2021) Special Section: COVID-19 and Cancer, [online], available: https://www.cancer.org/research/cancer-facts-statistics/all-cancer-facts-figures/cancer-facts-figures-2022.html [accessed 3 December 2022].
[6] European Commission. (2022). European Cancer Information System: 21% increase in new cancer cases by 2040, [online], available: https://joint-research-centre.ec.europa.eu/jrc-news/european-cancer-information-system-21-increase-new-cancer-cases-2040-2022-03-16_en [accessed 30 November 2022].
[7] Clatici, V.G., Voicu, C., Voaides, C., Roseanu, A., Icriverzi, M. and Jurcoane, S. (2018) Diseases of Civilization – Cancer, Diabetes, Obesity and Acne – the Implication of Milk, IGF-1 and mTORC1. MAEDICA – a Journal of Clinical Medicine, [online], 13 (4), pp. 273-281, available: ResearchGate GmbH [accessed 3 December 2022].
[8] Advanced Accelerator Applications, A Novartis Company. (2022). Nuclear medicine, [online], available: https://www.adacap.com/nuclear-medicine/ [accessed 3 December 2022].
[9] Advanced Accelerator Applications, A Novartis Company. (2022). Our Focus, [online], available: https://www.adacap.com/our-focus/ [accessed 16 January 2023].
[10] The Johns Hopkins University, The Johns Hopkins Hospital, and Johns Hopkins Health System. (2019). Nuclear medicine, [online], available: https://www.hopkinsmedicine.org/health/treatment-tests-and-therapies/nuclear-medicine [accessed 3 December 2022].
[11] U.S. Department of Health & Human Services. (2021). Radiation in healthcare: Nuclear medicine, [online], available: https://www.cdc.gov/nceh/radiation/nuclear_medicine.htm [accessed 3 December 2022].
[12] Advanced Accelerator Applications, A Novartis Company. (2022). Leading innovation for patients, [online], available: https://www.adacap.com/our-products/ [accessed 3 December 2022].
[13] Youth STEM 2030 CIC. (2022). The Role of Radioisotopes in Medical Diagnostic Procedures, [online], available: https://www.youthstem2030.org/youth-stem-matters/read/the-role-of-radioisotopes-in-medical-diagnostic-procedures#:~:text=The%20most%20common%20radioisotopes%20used,%2C%20or%20lungs%20%5B6%5D [accessed 3 December 2022].
[14] Curium. Life Forward. (2021). Products, [online], available: https://www.curiumpharma.com/products/european-products/ [accessed 3 December 2022].
[15] Figure 1. Wikimedia. (2007). File: EM Spectrum Properties edit.svg. Based off of File: EM Spectrum3-new.jpg by NASA. [online], available: https://commons.wikimedia.org/wiki/File:EM_Spectrum_Properties_edit.svg [accessed 3 December 2022].
[16] Stokowski, L.A. (2006). FUNDAMENTALS OF PHOTOTHERAPY FOR NEONATAL JAUNDICE. Advances in Neonatal Care, [online], 6 (6), pp. 303–312, available: https://doi.org/10.1016/j.adnc.2006.08.004 [accessed 3 December 2022].
[17] A U.S. Department of Energy National Laboratory Managed by the University of California. (2018). Light and Infrared Radiation, [online], available: https://ehs.lbl.gov/resource/documents/radiation-protection/non-ionizing-radiation/light-and-infrared-radiation/#:~:text=Prolonged%20exposure%20to%20IR%20radiation,eye%2C%20swelling%2C%20or%20hemorrhaging [accessed 3 December 2022].
[18] Cho, S., Shin, M.H., Kim, Y.K., Seo, J., Lee, Y.M., Park, C. and Chung, J.H. (2009). Effects of Infrared Radiation and Heat on Human Skin Aging in vivo. Journal of Investigative Dermatology Symposium Proceedings, [online], 14 (1), pp. 15–19, available: https://doi.org/10.1038/jidsymp.2009.7 [accessed 3 December 2022].
[19] U.S. Department of Health & Human Services. (2021). Radiation from the Earth (Terrestrial Radiation), [online], available: https://www.cdc.gov/nceh/radiation/terrestrial.html [accessed 3 December 2022].
[20] Kanu, A.M., Comfort, L.L., Guilderson, T.P., Cameron-Smith, P.J., Bergmann, D.J., Atlas, E.L., Schauffler, S. and Boering, K.A. (2016). Measurements and modelling of contemporary radiocarbon in the stratosphere. Geophysical Research Letters, [online], 43 (3), pp. 1399–1406, available: https://doi.org/10.1002/2015gl066921 [accessed 3 December 2022].
[21] Radiation Dosimetry. (2019). What is Carbon-14 – Production – Properties – Decay – Definition, [online], available: https://www.radiation-dosimetry.org/what-is-carbon-14-production-properties-decay-definition/ [accessed 3 December 2022].
[22] Abdallah, A.M., Mohery, M., Yaghmour, S.J. and Alddin, S.H. (2012). Radon exhalation and natural radiation exposure in low ventilated rooms. Radiation Physics and Chemistry, [online], 81 (11), pp. 1710-1714, available: Elsevier B.V. [accessed 3 December 2022].
[23] Mirion Technologies, Inc. (2015). Types of ionizing radiation, [online], available: https://www.mirion.com/learning-center/radiation-safety-basics/types-of-ionizing-radiation [accessed 3 December 2022].
[24] Venkatesh, M. and Kang, K.W. (2021) Encyclopedia of Nuclear Energy. Medicine: Radionuclides Used in Nuclear Medicine. ed., pp. 236–260, Elsevier B.V. [online] available: https://www.sciencedirect.com/science/article/pii/B978012819725700177X [accessed 3 December 2022].
[25] BBC. (2022). Radioactive emissions, [online], available: https://www.bbc.co.uk/bitesize/guides/z2qq4qt/revision/5#:~:text=The%20half-life%20of%20a%20radioactive%20isotope%20is%20the,plutonium-241%20has%20a%20half-life%20of%20only%2014.4%20years [accessed 3 December 2022].
[26] Wu, T., Chiu, H., Yu, J., Cautela, M.P., Sarmento, B., das Neves, J., Catala, C., Pazos-Perez, N., Guerrini, L., Alvarez-Puebla, R.A., Vranješ-Đurić, S. and Ignjatović, N.L. (2018). Nanotechnologies in Preventive and Regenerative Medicine. Chapter 1 – Nanotechnologies for early diagnosis, in situ disease monitoring, and prevention. ed., Elsevier B.V. [online] available: [accessed 3 December 2022].
[27] Kasperek-Nowakiewicz, R. (2020). Radiofarmaceutyki w lecznictwie. Aptekarz Polski. Pismo Naczelnej Izby Aptekarskiej, [online], 14 May, available: https://www.aptekarzpolski.pl/wiedza/radiofarmaceutyki-w-lecznictwie%ef%bb%bf/ [accessed 3 January, 2023].
[28] Vermeulen, K., Vandamme, M., Bormans, G. and Cleeren, F. (2019). Design and Challenges of Radiopharmaceuticals. Seminars in Nuclear Medicine, [online], 49 (5), pp. 339–356, available: Elsevier B.V. [accessed 3 December 2022].
[29] Figure 3. National Cancer Institute (NCI). (2020). Radiopharmaceuticals. [online], available: https://visualsonline.cancer.gov/details.cfm?imageid=12620 [accessed 10 January 2023].
[30] National Cancer Institute at the National Institutes of Health. (2013). Radium-223 Improves Survival in Patients with Advanced Prostate Cancer, [online], available: https://www.cancer.gov/types/prostate/research/radium-223-improves-survival#:~:text=Radium-223%20is%20a%20%E2%80%9Ccalcium%20mimetic%E2%80%9D%20that%2C%20like%20calcium%2C,such%20as%20areas%20where%20bone%20metastases%20are%20forming [accessed 3 December 2022].
[31] American Cancer Society. (2019). Radioactive Iodine (Radioiodine) Therapy for Thyroid Cancer, [online], available: https://www.cancer.org/cancer/thyroid-cancer/treating/radioactive-iodine.html [accessed 3 December 2022].
[32] Future US, Inc. (2019). Why Did People Take Iodine Pills After Chernobyl Exploded?, [online], available: https://www.livescience.com/65634-chernobyl-explosion-nuclear-disaster-iodine.html [accessed 3 December 2022].
[33] Strebhardt, K. and Ullrich, A. (2008) Paul Ehrlich’s magic bullet concept: 100 years of progress. Nature Reviews Cancer, [online], 8 (6), pp. 473–480, available: https://doi.org/10.1038/nrc2394 [accessed 3 December 2022].
[34] U.S. Food & Drug Administration. (2018). What is Gene Therapy?, [online], available: https://www.fda.gov/vaccines-blood-biologics/cellular-gene-therapy-products/what-gene-therapy#footnote1 [accessed 3 January 2023].
Bibliography
[1] Advanced Accelerator Applications, A Novartis Company. (2020). Theragnostic Platform, [online], available: https://www.adacap.com/theragnostic-platform/ [accessed: 3 December 2022].
[2] Beaumont Health. (2022). Side Effects of Radiation, [online], available: https://www.beaumont.org/treatments/side-effects-of-radiation#:~:text=Short-term%20side%20effects%20of%20radiation%20include%201%20skin,radiation%20and%20chemotherapy%20are%20given%20together%20More%20items [accessed: 4 January 2023].
[3] Klaris, G. (2016) The Future of Nuclear Medicine. Stanford University. Submitted as coursework for PH241, Stanford University, Winter 2016, [online], available: http://large.stanford.edu/courses/2016/ph241/klaris2/ [accessed: 3 December 2022].
[4] McKesson Medical-Surgical Inc. (2021) The future of nuclear medicine: Artificial intelligence, theranostics & beyond, [online], available: https://mms.mckesson.com/resources/patient-care-management/the-future-of-nuclear-medicine-artificial-intelligence-theranostics-beyond [accessed: 3 December 2022]
[5] NASA. (2023). The Electromagnetic Spectrum Video Series & Companion Book, [online], available: https://science.nasa.gov/ems/ [accessed 6 January 2023].
[6] Nuclear Power. (2023). Alpha, Beta and Gamma Radiation, [online], available: https://www.nuclear-power.com/nuclear-power/reactor-physics/atomic-nuclear-physics/radiation/alpha-beta-and-gamma-radiation/ [accessed 6 January 2023].
[7] U.S. Department of Health & Human Services. (2021). Radiation from Space (Cosmic Radiation), [online], available: https://www.cdc.gov/nceh/radiation/cosmic.html [accessed 3 December 2022].
[8] U.S. Department of Health & Human Services. (2021). The Electromagnetic Spectrum: Ionizing Radiation, [online], available: https://www.cdc.gov/nceh/radiation/ionizing_radiation.html [accessed 3 December 2022].
Author: Magdalena Ciuchta, Junior Life Science Consultant, KVALITO
KVALITO is a strategic partner, global quality and compliance service, and network for regulated industries. To find out more, please visit us at www.kvalito.ch. If you would like to benefit from KVALITO’s expert services, please email us at contact@kvalito.ch. Are you looking for an exciting and challenging position as a consultant, or are you an ambitious student/graduate looking for an internship? Please send your complete application to recruiting@kvalito.ch.